Things People Avoid Studying
Eugene Serebryany
Department of Chemistry and Chemical Biology
Harvard University, Cambridge MA 02138
evgeny_serebryany@fas.harvard.edu
What drew me to Jon as a mentor were his keen strategic insights in three areas. First, he, together with Cammie and the generations of their trainees, had optimized the lab for scientific productivity and impact far beyond its modest size in its final years of operation. Second, he shared his impressive scientific intuition freely and frequently. Third, and quite striking to me as a young graduate student, were his critical insights into what people in the life sciences avoided studying and why.
Things People Avoid Studying (TPAS, for short) are processes or phenomena typically described as non-specific, non-ordered, non-generalizable, non-urgent, or otherwise not warranting attention. In reality, they are often none of the above – but studying them is inconvenient, unconventional, or both. Jon, however, is always willing to entertain the scientifically contrarian view. This is a trait I share. Prior to joining the lab, I had spent four years as an undergraduate in Elsa Yan’s group at Yale working out the mechanism of negative receptor/ligand binding cooperativity; most biochemistry courses either ignore this phenomenon or teach that it does not occur. In the protein folding field, Jon taught me, TPAS have included: misfolding pathways and aggregated structures beyond the amyloid case; disulfides stable or transient; and the role of kinetic traps and kinetic stability.
Disulfides in particular have become a recurring theme for me. Even in my undergraduate work, the key to elucidating the mechanism of negative cooperativity was the removal of a single cross-interface disulfide in a large dimeric receptor domain, thus setting up a monomer-dimer equilibrium.1 In the King lab, I focused on the aggregation pathways of oxidation-mimicking Trp variants of human γD-crystallin,2 revealing another unusual phenomenon: the highly stable, aggregation-resistant WT protein drove a destabilized but still well folded variant into an aggregated state without itself aggregating3 (Figure 1). We thought of this as an “inverse prion” effect (a term inspired by the work of Susan Lindquist, who served initially as my co-advisor). In subsequent studies,4,5 we showed that this effect is mediated by transfer of disulfide bonds from a redox-active site on the WT protein to a non-native site in the W42Q variant, which locked a misfolded conformer prone to non-amyloid aggregation. Most recently, as a postdoc in the Shakhnovich group at Harvard, I drew inspiration from the disulfide chemistry of the lens crystallins to devise a high-throughput disulfide scanning (HTDS) methodology that aims to systematically discover protein conformations, including non-native conformations, and link them to phenotypic effects.6
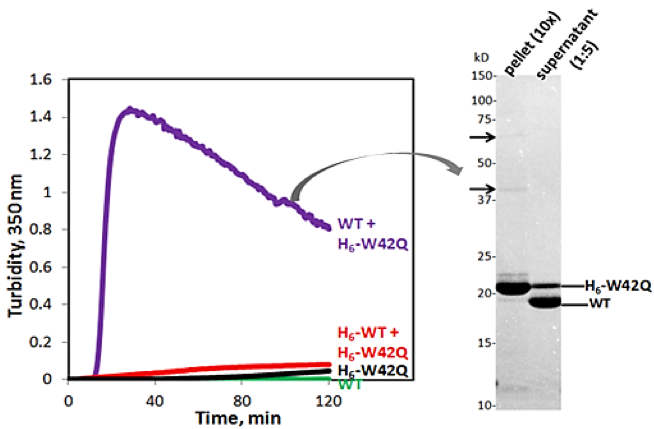
Figure 1: WT/mutant interaction drives aggregation of W42Q HγD. Turbidity kinetic traces of His-tagged H6-W42Q at 1 mg/ml with WT or H6-WT at 4 mg/ml. H6-W42Q with filtrate from WT is in black. SDS-PAGE of the turbid mixture shows only mutant protein is in the pellet fraction, while the WT remains fully soluble. Arrows indicate minor bands consistent with trimers and dimers of W42Q. Adapted from Ref. 3. |
A hallmark of all my Ph.D. years in the King lab was collaboration. As the lab grew smaller, the collaborations became both more important and more numerous. Jon welcomed visiting researchers from the Quintanar and Amero labs in Mexico City and sent me on a summer research visit to the Lampi and David labs at the Oregon Health and Science University. With his support, I initiated collaborations with the Cissé lab in MIT Physics, the Nolan lab in MIT Chemistry, the Drennan lab in MIT Biology, and the Shakhnovich lab in Harvard’s Chemistry and Chemical Biology department. We worked to ensure that the crystallin research the King lab had advanced so productively for many years would be transferred and carried forward elsewhere. In the end, Liliana Quintanar took the metal/crystallin research direction back to Cinvestav in Mexico while I brought the work on oxidative aggregation of γD-crystallin to the Shakhnovich lab. Other researchers and groups have since joined in, the National Eye Institute has provided funding, and these research directions continue.
TPAS abound in biomedical science. For understandable reasons, researchers, funders, and policymakers tend to focus on diseases that are especially deadly, especially frightening (e.g., due to their emergent nature or rapid progress), cause disproportionally large economic dislocation, or have no available treatment. Cataract falls into none of those categories. It does not directly kill though can certainly contribute to accidents and poor quality of life. It is so common that many people are resigned to it as simply a part of getting older. It develops slowly, and it afflicts mostly those who are retired or close to retirement. And cataract surgery is a long-established, mature industrial and medical field in the developed world. No sci-fi books are likely ever to mention a cataract cure or prophylactic. Some classmates gently teased me for studying such an undramatic disease.
Yet, there are moral, scientific, and practical reasons for taking this relatively less-traveled scientific path. Cataract afflicts so many that even a small reduction in its burden would tangibly improve millions of lives. Though surgery is usually successful, medical systems in the Global South can rarely afford to offer it, leaving most of the world’s cataract patients to go blind.7,8 Scientifically, cataract has much in common with deadlier pathologies of aging: oxidative damage and aberrant PTMs, protein misfolding and aggregation, and tissue degeneration. The eye lens is a much simpler tissue than, say, the brain and thus affords a better chance that biophysical research will translate into medical insights. In the words of a JBC reviewer of one of our crystallin papers, “this is one of those instances in which a careful biochemical-biophysical study provides direct key information of a biological (and human health) important problem.” I am proud to have made that sort of contribution. The remarkable biochemistry of the lens has also inspired new approaches and technologies,9-14 including, in my case, HTDS.6 Finally, the lens crystallin biophysics community, which Jon has played a very important part in building and continuing, is collegial, enthusiastic, and supportive. Without corrupting levels of public expenditure or glory, it continues to produce important, exciting research15-20 for the love of it.
1 Serebryany, E., Folta-Stogniew, E., Liu, J. & Yan, E. C. Y. Homodimerization enhances both sensitivity and dynamic range of the ligand-binding domain of type 1 metabotropic glutamate receptor. Febs Letters 590, 4308-4317, doi:10.1002/1873-3468.12473 (2016).
2 Serebryany, E. et al. Aggregation of Trp > Glu point mutants of human gamma-D crystallin provides a model for hereditary or UV-induced cataract. Protein Sci. 25, 1115-1128, doi:10.1002/pro.2924 (2016).
3 Serebryany, E. & King, J. A. Wild-type human gammaD-crystallin promotes aggregation of its oxidation-mimicking, misfolding-prone W42Q mutant. J. Biol. Chem. 290, 11491-11503, doi:10.1074/jbc.M114.621581 (2015).
4 Serebryany, E. et al. An Internal Disulfide Locks a Misfolded Aggregation-prone Intermediate in Cataract-linked Mutants of Human γD-Crystallin. J. Biol. Chem. 291, 19172-19183 (2016).
5 Serebryany, E., Yu, S., Trauger, S. A., Budnik, B. & Shakhnovich, E. I. Dynamic disulfide exchange in a crystallin protein in the human eye lens promotes cataract-associated aggregation. J. Biol. Chem. 293, 17997-18009, doi:10.1074/jbc.RA118.004551 (2018).
6 Serebryany, E. & Shakhnovich, E. High-Throughput Disulfide Scanning of In Vivo Protein Conformations. Biophysical Journal 120, 116A-116A (2021).
7 Wang, W. et al. Cataract Surgical Rate and Socioeconomics: A Global Study. Investigative Ophthalmology & Visual Science 57, 5872-5881, doi:10.1167/iovs.16-19894 (2016).
8 Organization, W. H. Visual Impairment and Blindness, <http://www.who.int/en/news-room/fact-sheets/detail/blindness-and-visual-impairment> (2014).
9 Garcia-Manyes, S. et al. Single-molecule Force Spectroscopy Predicts a Misfolded, Domain-swapped Conformation in human gammaD-Crystallin Protein. J Biol Chem 291, 4226-4235, doi:10.1074/jbc.M115.673871 (2016).
10 Alperstein, A. M., Ostrander, J. S., Zhang, T. Q. O. & Zanni, M. T. Amyloid found in human cataracts with two-dimensional infrared spectroscopy. Proc. Natl. Acad. Sci. U. S. A. 116, 6602-6607, doi:10.1073/pnas.1821534116 (2019).
11 Aryal, S. et al. MS/MS in silico subtraction-based proteomic profiling as an approach to facilitate disease gene discovery: application to lens development and cataract. Human Genetics 139, 151-184, doi:10.1007/s00439-019-02095-5 (2020).
12 Boatz, J. C., Whitley, M. J., Li, M. Y., Gronenborn, A. M. & van der Wel, P. C. A. Cataract-associated P23T gamma D-crystallin retains a native-like fold in amorphous-looking aggregates formed at physiological pH. Nat. Commun. 8, 10, doi:10.1038/ncomms15137 (2017).
13 Bierma, J. C. et al. Controlling Liquid Liquid Phase Separation of Cold-Adapted Crystallin Proteins from the Antarctic Toothfish. Journal of Molecular Biology 430, 5151-5168, doi:10.1016/j.jmb.2018.10.023 (2018).
14 Makley, L. N. et al. Pharmacological chaperone for alpha-crystallin partially restores transparency in cataract models. Science 350, 674-677, doi:10.1126/science.aac9145 (2015).
15 Berry, V. et al. Inherited cataracts: molecular genetics, clinical features, disease mechanisms and novel therapeutic approaches. British Journal of Ophthalmology 104, 1331-1337, doi:10.1136/bjophthalmol-2019-315282 (2020).
16 Ainsbury, E. A. & Barnard, S. G. R. Sensitivity and latency of ionising radiation-induced cataract. Experimental Eye Research 212, doi:10.1016/j.exer.2021.108772 (2021).
17 Fan, X. J. & Monnier, V. M. Protein posttranslational modification (PTM) by glycation: Role in lens aging and age-related cataractogenesis. Experimental Eye Research 210, doi:10.1016/j.exer.2021.108705 (2021).
18 Schey, K. L., Wang, Z., Friedrich, M. G. & Truscott, R. J. W. New insights into the mechanisms of age-related protein-protein crosslinking in the human lens. Experimental Eye Research 209, doi:10.1016/j.exer.2021.108679 (2021).
19 Roskamp, K. W., Paulson, C. N., Brubaker, W. D. & Martin, R. W. Function and Aggregation in Structural Eye Lens Crystallins. Accounts of Chemical Research 53, 863-874, doi:10.1021/acs.accounts.0c00014 (2020).
20 Serebryany, E., Thorn, D. C. & Quintanar, L. Redox chemistry of lens crystallins: A system of cysteines. Experimental Eye Research 211, doi:10.1016/j.exer.2021.108707 (2021).